The footprint of column collapse regimes on pyroclastic flow temperatures and plume heights
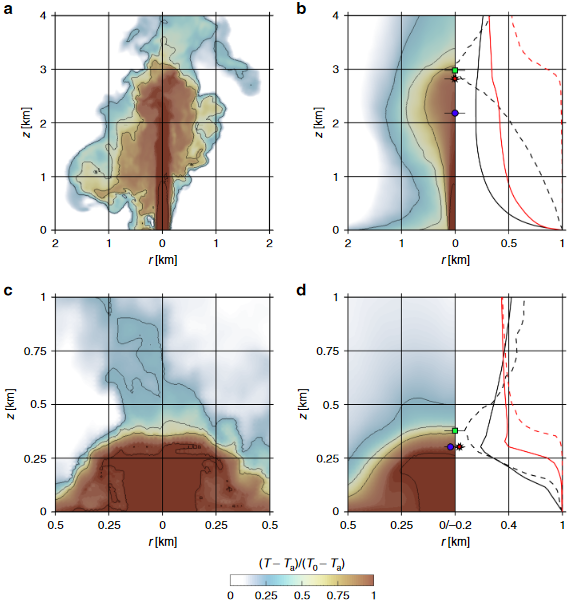
Trolese M., M. Cerminara, T. Esposti Ongaro, and G. Giordano (2019).
Nature Communications, 10/2476, doi: 10.1038/s41467-019-10337-3.
Abstract
The gravitational collapse of eruption columns generates ground-hugging pyroclastic density currents (PDCs) with highly variable temperatures, high enough to be a threat for communities surrounding volcanoes. The reasons for such great temperature variability are debated in terms of eruptive versus transport and emplacement processes. Here, using a three-dimensional multiphase model, we show that the initial temperature of PDCs linearly correlates to the percentage of collapsing mass, with a maximum temperature decrease of 45% in the case of low percentages of collapse (10%), owing to an efficient entrainment of air into the jet structure. Analyses also demonstrate that column collapse limits the dispersal capabilities of volcanic plumes, reducing their maximum height by up to 45%. Our findings provide quantitative insights into the mechanism of turbulent mixing, and suggest that temperatures of PDC deposits may serve as a marker for determining column collapse conditions, which are of primarily importance in hazard studies.
Devi effettuare l'accesso per postare un commento.